- Chalmers, Gothenburg, Sweden
- Columbia University, USA
- Duke University | Pratt School of Engineering, USA
- Hong Kong University of Science and Technology (China)
- Linköpings University, Sweden
- Manchester University, UK
- Monash Centre for Atomically Thin Materials (MCATM), Australia
- National University of Singapore
- Ohio State University, USA
- Penn State Materials Research Institute – USA
- Rice University, Houston TX, USA
- Seoul National University, South Korea
- Stanford University Engineering, USA
- The Hong Kong Polytechnic University (HK)
- Tohoku University – Advanced Institute for Materials Research
- Ulsan National Institute of Science and Technology
- University of Cambridge, UK
- University of Dublin, Trinity College
- University of Exeter, UK
- University of Illinois, Mason Group, USA
- University of Massachusetts, Francis College of Engineering, USA
- University of Missouri, USA
- University of Siegen, Germany
- University of Texas, Austin
- University of Wollongong, Australia
Category: Graphene
Graphene Sensors for Ammonia Detection
Graphene is the thinnest, strongest material known to man. Graphene is a single layer of carbon atoms, tightly bound in a honeycomb crystal lattice that’s the basic structural element of industrial and manufacturing applications of carbon, including graphite, charcoal, and carbon nanotubes.
Graphene applications include lightweight, thin, flexible electric and photonics circuits, solar cells, as well as an input for products with applications in medical, chemical, and industrial processes.
MIT and Graphenea have developed an array of graphene sensors for sensitive and selective detection of ammonia. The array consists of 160 graphene pixels, allowing large statistics that result in improved sensing performance. The sensors are extensively tested for various real-life operational conditions, which is a step forward to practical use.
The sensors are built by attaching porphyrins, a class of organic molecules, to the graphene surface. Porphyrins are particularly well-matched to graphene sensors because they provide excellent sensitivity while producing minimal perturbation to graphene’s outstanding electrical properties. When ammonia molecules attach to porphyrins, the compound becomes a strong dipole that changes electrical properties of the graphene. This electrical change is detected as a sign of the presence of ammonia.
The graphene sensor array is designed as an insertable chip for use in conjunction with a custom readout system, which is connected to a computer via USB.
Together with specialized data acquisition software, the system can perform rapid high-quality readout of data from hundreds of sensors, which is a major improvement over previous prototype graphene sensor devices.
The ability to monitor a large number of sensors allows monitoring performance variations and reproducibility and subsequent improvement of sensor array performance.Importantly, the graphene sensors are shown to be selective – they respond to ammonia but not to other molecules such as water, hexane, ethanol, chloroform, or acetonitrile. The sensor electrical properties change by as much as 8% for ammonia concentrations of 160 ppm, although concentrations as small as 20 ppm were successfully detected. These sensors are 4 times more sensitive to ammonia then pristine graphene sensors reported earlier.
Although quantification was performed in a dry nitrogen atmosphere, the specificity and sensitivity to ammonia were shown to remain even in an air environment, which enables robust performance in real use conditions. The variations in performance between sensor pixels were small, allowing reproducibility and mass production.
The findings were published in the journal ACS Applied Materials & Interfaces. The work opens doors for further quality engineering and advancement of this sensing technology.
What is Difference among Activated Carbon, Graphite, Graphene and Fullerence ?
Charcoal and Activated Carbons
Graphite
The chemical bonds in graphite are similar in strength to those found in diamond. However, the lattice structure of the carbon atoms contributes to the difference in hardness of these two compounds; graphite contains two dimensional lattice bonds, while diamond contains three dimensional lattice bonds. The carbon atoms within each layer of graphite contain weaker intermolecular bonds. This allows the layers to slide across each other, making graphite a soft and malleable material.
Various studies have demonstrated that graphite is an excellent mineral with several unique properties. It conducts heat and electricity and retains the highest natural strength and stiffness even in temperatures exceeding 3600°C. This material is self-lubricating and is also resistant to chemicals.
Although there are different forms of carbon, graphite is highly stable under standard conditions. Depending upon its form, graphite is utilized for a wide range of applications.
Graphene
What is the Difference between Graphite and Graphene ?
Since graphite has a planar structure, its electronic, acoustic, and thermal properties are highly anisotropic. This means, phonons pass much more easily along the planes than they do when trying to pass via the planes. However, graphene has very high electron mobility and, like graphite, is a good electrical conductor, due to the occurrence of a free pi (p) electron for each carbon atom.
However, graphene has much higher electrical conductivity than graphite, due to the occurrence of quasiparticles, which are electrons that function as if they have no mass and can travel long distances without scattering. In order to fully realize this high level of electrical conductivity, doping needs to be carried out to overcome the zero density of states which can be visualized at the Dirac points of graphene.
Fullerence
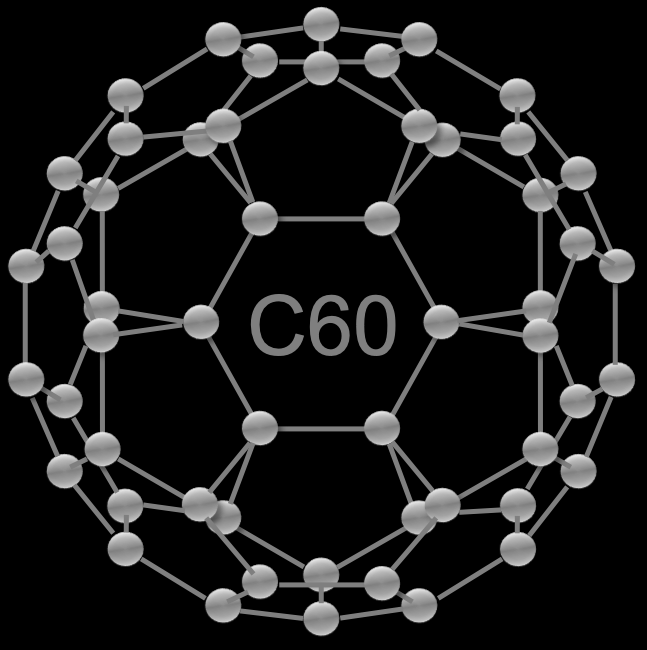
A fullerene is an allotrope of carbon whose molecule consists of carbon atoms connected by single and double bonds so as to form a closed or partially closed mesh, with fused rings of five to seven atoms. The molecule may be a hollow sphere, ellipsoid, tube, or many other shapes and sizes. Graphene (isolated atomic layers of graphite), which is a flat mesh of regular hexagonal rings, can be seen as an extreme member of the family.
Fullerenes with a closed mesh topology are informally denoted by their empirical formula Cn, often written Cn, where n is the number of carbon atoms. However, for some values of n there may be more than one isomer.
The family is named after buckminsterfullerene (C60), the most famous member, which in turn is named after Buckminster Fuller. The closed fullerenes, especially C60, are also informally called buckyballs for their resemblance to the standard ball of association football (“soccer”). Nested closed fullerenes have been named bucky onions. Cylindrical fullerenes are also called carbon nanotubes or buckytubes. The bulk solid form of pure or mixed fullerenes is called fullerite.
Fullerenes had been predicted for some time, but only after their accidental synthesis in 1985 were they detected in nature and outer space. The discovery of fullerenes greatly expanded the number of known allotropes of carbon, which had previously been limited to graphite, diamond, and amorphous carbon such as soot and charcoal. They have been the subject of intense research, both for their chemistry and for their technological applications, especially in materials science, electronics, and nanotechnology.
What is Carbon Nanotube Chip ?
Carbon nanotubes (CNTs) are tubes made of carbon with diameters typically measured in nanometers. Carbon nanotubes often refer to single-wall carbon nanotubes (SWCNTs) with diameters in the range of a nanometer. Single-wall carbon nanotubes are one of the allotropes of carbon, intermediate between fullerene cages and flat graphene.
Although not made this way, single-wall carbon nanotubes can be idealized as cutouts from a two-dimensional hexagonal lattice of carbon atoms rolled up along one of the Bravais lattice vectors of the hexagonal lattice to form a hollow cylinder. In this construction, periodic boundary conditions are imposed over the length of this roll up vector to yield a helical lattice of seamlessly bonded carbon atoms on the cylinder surface.
Carbon nanotubes also often refer to multi-wall carbon nanotubes (MWCNTs) consisting of nested single-wall carbon nanotubes weakly bound together by van der Waals interactions in a tree ring-like structure. If not identical, these tubes are very similar to Oberlin, Endo, and Koyama’s long straight and parallel carbon layers cylindrically arranged around a hollow tube. Multi-wall carbon nanotubes are also sometimes used to refer to double- and triple-wall carbon nanotubes.
Carbon nanotubes can also refer to tubes with an undetermined carbon-wall structure and diameters less than 100 nanometers. Such tubes were discovered in 1952 by Radushkevich and Lukyanovich.
While nanotubes of other compositions exist, most research has been focused on the carbon ones. Therefore, the “carbon” qualifier is often left implicit in the acronyms, and the names are abbreviated NT, SWNT, and MWNT.
The length of a carbon nanotube produced by common production methods is often not reported, but is typically much larger than its diameter. Thus, for many purposes, end effects are neglected and the length of carbon nanotubes is assumed infinite.
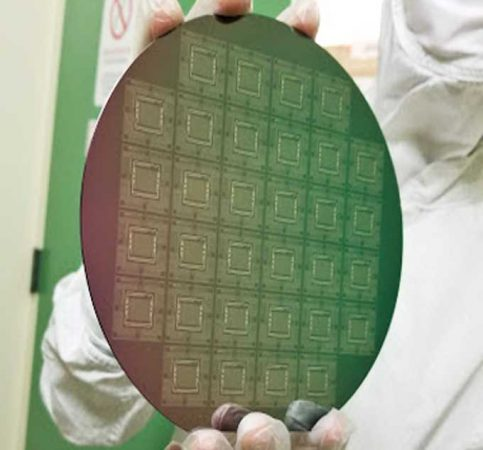
A carbon nanotube computer is a computer built entirely using carbon nanotubes (CNT) based transistors. Researchers from Stanford University said that they had successfully built a carbon nanotube computer and their research paper published on 25 September 2013 in the journal Nature.
They named their first carbon nanotube computer Cedric. It has a one-bit processor containing just 178 transistors.
In 2019, a team at the Massachusetts Institute of Technology created the 16-bit processor called RV16X-NANO. With 14 000 transistors (compared to only hundreds in the first CNT computer made in 2013) it is the largest computer chip yet to be made from carbon nanotubes. It was able to execute a “Hello, World!” program with a message: “Hello, world! I am RV16XNano, made from CNTs”. It is based on the RISC-V instruction set and runs standard 32-bit instructions on 16-bit data and addresses.
Computer chips from carbon nanotubes, not silicon, mark a milestone
A new type of computing chip could be a game-changer. That’s because its transistors are not made of silicon. Transistors are tiny electronic switches that together perform calculations. A new prototype uses carbon nanotubes. It is not yet as speedy or as small as the silicon devices found in today’s computers, phones and more. But these new computer chips may one day give rise to electronics that are faster and use less energy.
Researchers describe their advance in the August 29 Nature.
This is “a very important milestone in the development of this technology,” observes Qing Cao. He’s a materials scientist at the University of Illinois at Urbana-Champaign. He was not involved in the work.
The heart of every transistor is a semiconductor component. It’s usually made of silicon. This element can act like an electrical conductor. It also can act like an insulator. This lets a transistor have an “on” and an “off” state. When on, current flows through the semiconductor; when off, it doesn’t. And this on/off state is what encodes the 1s and 0s of digital computer data.
Max Shulaker is an electrical engineer. He works at the Massachusetts Institute of Technology in Cambridge. “We used to get exponential gains in computing every single year,” he says. Computer engineers were able to do so by building smaller and faster silicon transistors. But now, he says, “performance gains have started to level off.” Silicon transistors can’t get much smaller and more efficient than they already are.
Carbon nanotubes, though, are almost as thin as an atom. And they ferry electricity well. As a result, they make better semiconductors than silicon. In principle, carbon nanotube processors could run three times faster than silicon ones. And they would consume about one-third as much energy as silicon processors, Shulaker says. But until now, carbon nanotubes have proved too finicky to use in complex computing systems.
Carbon computing
One issue comes when a network of carbon nanotubes is deposited onto a computer chip wafer. At that point, the tubes tend to bunch into lumps. This prevents the transistor from working. It’s “like trying to build a brick patio, with a giant boulder in the middle of it,” Shulaker says. His team solved that problem. They spread nanotubes on a chip. Then they used vibrations to gently shake unwanted bundles off the layer of nanotubes.
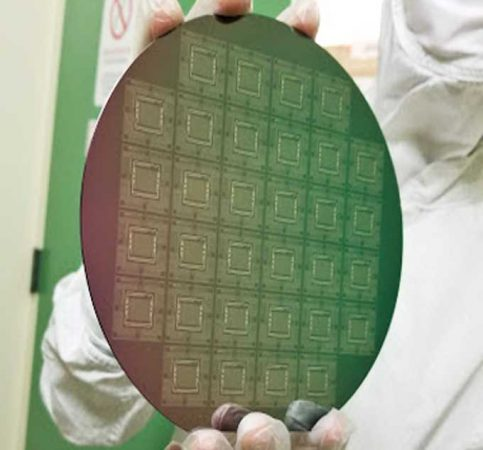
The team also faced another problem. Each batch of carbon nanotubes contains about 0.01 percent metallic nanotubes. Metallic nanotubes can’t properly flip between conductive and insulating. So these tubes can muddle a transistor’s readout.
Shulaker and colleagues searched for a workaround. To perform different kinds of operations on bits of data, transistors can be configured in various ways. The researchers looked at how metallic nanotubes affected different configurations. They found that defective nanotubes affected the function of some configurations more than others. This is similar to the way a missing letter can make some words illegible, but leave others mostly readable. So the researchers carefully designed the circuitry of their microprocessor. They avoided configurations that were most confused by metallic-nanotube glitches.
“One of the biggest things that impressed me about this paper was the cleverness of that circuit design,” says Michael Arnold. He’s a materials scientist at the University of Wisconsin–Madison. He was not involved in the work.
The resulting chip has more than 14,000 carbon-nanotube transistors. It executed a simple program to write the message, “Hello, world!” This is the first program that many newbie computer programmers learn to write.
The new chips are not yet ready to unseat silicon ones in modern electronics. Each carbon transistor is about a millionth of a meter across. Current silicon transistors are smaller. They are tens of billionths of a meter across. Each carbon-nanotube transistor in this prototype can flip on and off about a million times a second. Silicon transistors can flicker billions of times per second. That puts nanotube transistors on a par with silicon transistors of the 1980s.
Shrinking the nanotube transistors would help electricity zip through them with less resistance. That would allow the devices to switch on and off faster, Arnold says. They could also align the nanotubes in parallel, rather than using a randomly oriented mesh. This could increase the electric current through the transistors. That would further boost processing speeds.
What Are the Main Applications of Graphene ?
Graphene is a one-atom-thick sheet of carbon atoms arranged in a honeycomb-like pattern. Graphene is considered to be the world’s thinnest, strongest and most conductive material – of both electricity and heat. All of these properties are exciting researchers and businesses around the world – as graphene has the potential to revolutionize entire industries – in the fields of electricity, conductivity, energy generation, batteries, sensors and more.
Graphene is a disruptive technology; one that could open up new markets and even replace existing technologies or materials. It is when graphene is used both to improve an existing material and in a transformational capacity that its true potential can be realized.
Mechanical strength
Graphene is the world’s strongest material, and can be used to enhance the strength of other materials. Dozens of researchers have demonstrated that adding even a trace amount of graphene to plastics, metals or other materials can make these materials much stronger – or lighter (as you can use a smaller amount of material to achieve the same strength).
Such graphene-enhanced composite materials can find uses in aerospace, building materials, mobile devices, and many other applications.
The vast number of products, processes and industries for which graphene could create a significant impact all stems from its amazing properties.
No other material has the breadth of superlatives that graphene boasts, making it ideal for countless applications.
- It is many times times stronger than steel, yet incredibly lightweight and flexible.
- It is electrically and thermally conductive but also transparent.
- It is the world’s first 2D material and is one million times thinner than the diameter of a single human hair.
What is the Applications of Graphene ?
Transport, medicine, electronics, energy, defence, desalination; the range of industries where graphene research is making an impact is substantial.
And this is only the start. These are only the first steps. The potential of graphene is limited only by our imagination.
1. Biomedical
Graphene’s unique properties allow for ground-breaking biomedical applications. Targeted drug delivery; improved brain penetration; DIY health-testing kits and ‘smart’ implants.
-
- Graphene in Drug Delivery – Functionalized graphene can be used to carry chemotherapy drugs to tumors for cancer patients. Graphene based carriers targeted cancer cells better and reduced and decreased toxicity of the effected healthy cells. Drug delivery is not limited to cancer treatment, anti-inflammatory drugs have also been carried by graphene & chitosan combinations and yielded promising results.
- Graphene in Cancer Treatment – Graphene can also detect cancer cells in the early stages of the disease. Moreover, it can stop them from growing any further in many types of cancer by intervening the correct formation of the tumor or causing autophagy which leads to the death of cancer cells.
- Graphene in Gene Delivery – Gene delivery is a method used to cure some genetic diseases by bringing foreign DNA into cells. Graphene Oxide modified by Polyethyleneimine can be used for these purposes is expected to show low cytotoxicity, as it did in the drug delivery case.
- Graphene in Photothermal Therapy – Photothermal therapy (PTT) is a approach used to eliminate abnormal cells in the targeted area of the body by irradiating a special agent which creates heat capable of destructing those cells. Graphene oxide increases effectiveness of PTT by a number of ways. First, it can be used to carry chemotherapeutic drugs to the tumor cells while they are being exposed to PTT simultaneously. Combining chemo and PTT like this is more effective than using one of these approaches alone. A nanocomposite of reduced graphene oxide (QD-CRGO) can be used during PTT for bioimaging of the cancer cells. Moreover, in their research, a group of scientists from Texas Tech and Texas A&M University have shown that using graphene oxide functionalized with biocompatible porphyrin as a platform for PTT for brain cancer have killed more cancer cells than PTT alone, while giving no harm to the healthy cells.
- Graphene in Diabetes Monitoring – Scientists from the University of Bath have developed a blood glucose monitoring test which does not pierce the skin, unlike currently used finger prick tests. This patch, including a graphene sensor, is able to work on a small area containing at least one hair follicle. It detects the glucose by pulling it from the fluid present between the cells. This does not only end the painful methods of blood sugar monitoring, but is also expected to increase the accuracy of the results.
- Graphene in Dialysis – Graphene membranes are not only useful for the energy, nuclear and food industries. A group of researchers from MIT showed that graphene can be used to filter the blood from wastes, drugs and chemicals as well. Graphene’s superiority in this case is that it is 20 times thinner than traditional membranes which leads to significant decrease in the time spent in the dialysis for the patients.
- Graphene in Bone and Teeth Implantation – Hydroxyapatite, a form of calcium apatite, is a material used as a synthetic bone substitute for regenerated bone and dental tissues. Graphene, combined with Hydroxyapatite and Chitosan, have shown increase in the strength, corrosion resistance, flexibility and mechanical & osteogenic properties of the substitute when compared to HAp alone.
- Graphene in Tissue Engineering and Cell Therapy – Bones are not the only tissue graphene can cure. Certain forms of graphene were shown to be compatible with human osteoblasts and human mesenchymal cells, showing similar properties with the cells’ physiological microenvironment. Cells grown with this method demonstrated better growth, proliferation, and differentiation while being ineffective on the cells’ viability. Stem cells are especially important in tissue reengineering to improve the lives of people with neuronal disorders or neurodegenerative diseases.
- Graphene for the Brain – Mysteries about the brain haven’t been revealed completely yet. A graphene-based technology may allow scientists to uncover many of the unknowns by recording brains electrical activity. This new device is able to hear the frequencies below older technologies’ limits, and it doesn’t interfere with the functioning of the brain. Besides research on how the brain works, the technology can help the scientists to understand the reasons behind epilepsy seizures and develop treatments for the patients. Moreover, discovering more about the brain could lead to developing new Brain-Computer interfaces which are used in many areas including control of prosthetic limbs.
- Graphene in HIV Diagnosis – Despite all the improvements, there are many drawbacks on current HIV diagnosis methods. They can either detect the antibodies in the body nearly a month later the patient was infected, or they can detect the virus itself however these methods take some time to process themselves and more expensive when compared to the antibody method. A biosensor made of silicon or graphene, containing Gold Nanoparticles was developed by Spanish National Research Council, which targets p24, an antigen found on HIV. The new method can detect the virus only a week after being infected and at levels 100,000 times lower than what the current tests can notice. Moreover, results of the test are ready within 5 hours of being tested
- Graphene Biosensors – One of the advantages of graphene is its ability to detect minimal amounts of substances. Even a single molecule in a large volume can be detected with it. Biosensors made of graphene, graphene oxide or reduced graphene oxide show ultrasensitive properties when detecting DNA, ATP, dopamine, oligonucleotides, thrombin, and different atoms. There are several medical companies that already sell medical sensors made with graphene.
- Graphene Bactericide – Graphene is a magnificent bactericidal material as it avoids the generation of microorganisms, such as bacteria, viruses, and fungi, by damaging their cell membranes between its outer layers. When compared to different derivatives of Graphene, Graphene Oxide and reduced Graphene Oxide shows the best antibacterial effects. GO can also be used as a compound with silver nanoparticles to increase antibacterial properties even further.
- Graphene in Birth Control – Graphene has all the properties that is desired in a condom: it is flexible, extra strong and extremely thin. Researchers from Manchester University have worked on developing a “supercondom” made of graphene and latex combined. The research has received many funding, including one from Bill and Melinda Gates Foundation.
- Graphene in Deaf-Mute Communication – A group of Chinese scientists have developed a wearable, bio-integrated device that can translate sign language into text and spoken language. The device uses graphene’s incredible conductivity and flexibility properties.
- Graphene in Body Scans – Unlike X-rays, T-waves which can be used for body scanning are harmless to human body. However, there is a catch. T-waves, or THZ radiation, is hard to both detect and generate. The good news is, with the help of some modifications and other materials, CVD graphene can detect THZ radiation successfully. This will not only lead to safer body scans, but also incredibly faster internet in the future
2. Graphene-based composite materials
Graphene is a material with a huge amount of outstanding qualities; strength, flexibility, lightweight and conductivity.
-
- Graphene in Shoes – Graphene sneakers? Yes, although in this case it is not used purely, other composite materials take advantage of it. In fact, it is claimed that a sole made of pure graphene could last hundreds of years. University of Manchester and sports brand Inov-8 developed a shoe using graphene which increases the outsoles’ strength and flexibility properties by 50%. These shoes are more durable and absorbs the impacts which could damage the bones and joints.
- Graphene in Helmets – An ideal helmet would be strong, resistant to impact, durable, comfortable, and light. Graphene is incredibly strong, light, and flexible. It’s even used in bulletproof vests, so it can definitely resist impacts. With these properties, graphene is commercially used in motorbike helmets.
- Graphene in Tires – Graphene is also used to make smarter tires and sports bike components. Adding graphene to bike’s tires apparently increases the puncture resistance and velocity, decreases rolling resistance and makes them way lighter, stronger, faster, and more resilient.
- Graphene Clothes – The use of graphene fibers in fabrics offers antibacterial, and anti-static clothes which can preserve the heat and block UV. These fabrics can be used to create outdoor sportswear, pajamas for children that repel soil bacteria, or even household furniture to prevent the development of bacteria on its surface.
- Graphene Rackets – Graphene can improve the energy distribution and weight of the racket while increasing the service speed and stability. Tennis equipment manufacturer Head have already developed a series of commercially available racket enhanced with graphene, named “Graphene 360” which is already used by tennis stars like Novak Djokovic and Sascha Zverev.
- Graphene Electronic Tattoos and Fitness Tracking – Graphene Electronic Tattoo (GET) is developed by scientists at the University of Texas. First, they are more resistant to moisture, have a greater elasticity – with the ability to grow or shrink up to 40%, have a total thickness of 463 ± 30 nm, and have optical transparency of approximately 85%. They are like a second skin. These tattoos can be used to track heart rate, temperature, hydration levels, oxygen saturation and even the level of exposure to UV. Their application areas can vary from fitness tracking to medicine.
3. Electronics, Sensors, and Computers
Graphene has the potential to create the next-generation of electronics currently limited to sci-fi. Faster transistors; semiconductors; bendable phones and other electronics.
-
- Graphene UV Sensors – UV sensors are used for detecting dangerous levels of ultra-violet radiation which can lead to skin problems or even cancer. However, it is not the only use of UV sensors, they are used in the military, optical communication, and environmental monitoring as well. On its own, graphene may not present a high photoresponsivity but when it is combined with other materials, they create flexible, transparent, environmentally-friendly and low-cost UV sensors which will lead to technologies such as wearable electronics in the close future.
- Graphene Transistors – The new supertransistors, which replace silicon with graphene, can increase the speed of computers up to one thousand times when compared to current technology. Increasing speed of computers is a crucial step for many technologies to be able to improve, including but not limited to blockchain, simulations of the outer space, robots, and stock markets.
- Graphene in Waterproof Electronics – One of the main problems of electronic devices which people are afraid of is being dropped to water. Instead of covering the device with tight-fitted screws, graphene proposes a great solution for this problem. Engineers from Iowa State University print the circuits of the device with graphene flakes because graphene is transparent, strong and conducts electricity. Graphene flakes are arranged in a specific order and non-conductive binders are used to combine them which improved the conductivity. As in the most application areas, graphene again puts a great solution to this problem.
- Graphene in Wearable Electronics – Researchers are looking for new ways to power wearable devices. One of the outstanding ways is flexible batteries printed on a fabric with graphene. This enables people to wear their batteries and power their smartphones or other devices, literally. If this can be achieved, it will be an environmentally friendly and smart e-textile that can store energy. Carrying heavy power-banks or chargers will be history by the invention of this amazing idea.
- Graphene for Touchscreens – Indium tin oxide (ITO) is the commercial product used as transparent conductor of the smartphones, tablets, and computers. Researchers from the Rice University have developed a graphene-based thin film to be used in touchscreens. It is found that graphene-based thin film beats ITO and any other materials in terms of performance because it has lower resistance and higher transparency. Thus, Graphene is the new candidate material for the replacement of ITO.
- Graphene in Flexible Screens – The world of technology would be one of the great beneficiaries of the standardization of graphene as a material to incorporate in products such as smartphones or tablets. It would be the definitive step to advance in the world of smartphones. Recently, a Chinese company has produced a bendable smartphone with a graphene touch screen. Since one layer of graphene is strong, light, transparent and very conductive, it meets all the requirements for the production of smartphones. The smartphone of the Chinese company has the ability to wrap a twist completely, and it weighs only 200 grams which propose a perfect convenience for usage. However, production of graphene is expensive at an industrial scale relative to other materials used in smartphones. Researchers are looking for ways to produce graphene at lower costs. When this problem and some others are solved, old phones seem to be replaced by these flexible smartphones in the future.
- Graphene in Hard Drives and Memories – Usually, graphene is not considered magnetic, at least not in a controllable or useful way. In 2015, researchers from U.S. Naval Research Laboratory have found a way to turn graphene into a reliable and controllable electromagnetic material. If this innovation is used in hard drives, it is expected to have a capacity almost a million times greater than what we use today.
- Graphene in Elastic Robots – A team of researchers has developed a gel that is sensitive to near infrared light so that it could be used in numerous applications when creating flexible or elastic robotic parts. The snake-like robots created with this method are able to change its form without any forces from the outside. Their future applications can vary from search-and-rescue to medical operations.
- Graphene as a Superconductor – Scientists have discovered that graphene can also be used as a superconductive material. Two layers of Graphene can conduct the electron without any resistance. This can be accomplished by twisting these two layers of graphene at a ‘magic angle’ which is 1.1°. Most of the superconductive materials show their properties at temperatures close to absolute zero. Even High temperature superconductive materials relative to usual ones can work at around -140°C. In other words, these superconductive materials require a huge energy for cooling. If graphene can be used as a superconductive material at temperatures close to room temperature, there will be a huge revolution for many application areas.
- Graphene in Optoelectronics – Researchers are working on a new material for the optical communications since energy and power requirement increase as the time passes. A research conducted by the collaboration of different universities has shown that integrating graphene with silicon can beat current silicon photonic technology. How can it beat the current state of art? Because devices made by graphene are cheaper, simpler and work at high-scale wavelengths. Apparently, graphene will present a low-energy optical telecommunication and many other convenient optical systems.
- Graphene in Optical Sensors – Graphene has a lot of breakthroughs in industry and science owing to its super properties. Researchers tried to shrink the light to make optical sensors smaller. Recently, the Institute of Photonic Sciences (ICFO) in Barcelona, with the collaboration of Graphene Flagship team, conducted a study which explains the reduction of light down to just a single atom thick which is thought to be impossible by many researchers. This discovery will lead to a huge step in ultra-small optical sensors and switches.
- Graphene Security Sensors – One of the first practical and real applications of graphene was security labels. Instead of the bulky sensors that many stores use, the sensors made with graphene are smaller, more aesthetic, able to bend without creating a damage on the circuit, and cost only a couple cents per tag.
4. Energy Storage and Thermal Applications
Imagine fully charging a smartphone in seconds, or an electric car in minutes. That’s the power of graphene. Since graphene is the world’s thinnest material, it also extremely high surface-area to volume ratio. This makes graphene a very promising material for use in batteries and supercapacitors. Graphene may enable batteries and supercapacitors (and even fuel-cells) that can store more energy – and charge faster, too.
Graphene is the most heat conductive found to date. As graphene is also strong and light, it means that it is a great material for making heat-spreading solutions, such as heat sinks or heat dissipation films. This could be useful in both microelectronics (for example to make LED lighting more efficient and longer lasting) and also in larger applications – for example thermal foils for mobile devices. Huawei’s latest smartphones, for example, have adopted graphene-based thermal films.
-
- Graphene in Solar Cells – The idea of developing lighter, flexible and transparent solar cells has been around for a while but finding the material which has all the properties and able to carry the current was the issue. Indium Tin Oxide has been used because it was transparent, however it was not flexible therefore the cell had to remain stiff.In 2017, researchers from MIT have managed to apply Graphene successfully on a solar cell. When they compared the graphene solar cell with others made of Aluminum and Indium Tin Oxide, they saw that it was as good as the ITO cell, and a little worse than Al one in terms of current densities and power conversion efficiencies. However, it is expected for a transparent cell to perform lower than Aluminum-based, which is nontransparent.Although electrical properties were not a breakthrough, a solar cell that can be installed on any kind of surface (cars, clothes, paper, and cell phones, etc.) which is flexible and transparent was developed. Moreover, other scientists are trying to find out if graphene solar cells can generate energy from raindrops, which theoretically looks possible.
- Graphene Batteries – Graphene enhanced Li-ion batteries show incredible characteristics such as longer lifespan, higher capacity, and faster charging time as well as flexibility and lightness, so that it could be used in wearable electronics.
- Graphene in Nuclear Power Plants – Heavy water used in nuclear power plants to cool the reactors is both costly to produce and causes a million tons of CO2 emissions during production. Researchers from University of Manchester have discovered that there is a greener and low-cost method to produce heavy water: graphene membranes. Team leader Dr. Lozada-Hidalgo believes that this innovation is extremely important and its introduction to the nuclear industry will be soon even though this industry is usually skeptical about new technologies.
- Graphene in Thermoelectric – Seebeck effect is defined as a thermoelectric effect occurring when heat is applied to one of the two dissimilar electric conductors (or semiconductors) to move the electrons from the hot part to the cooler part and produce electricity. However, the energy generated by this method is really small, usually quantified by microvolts. Still, it is believed that it can be used to benefit from the heat generated by the engines, which is practically wasted. Graphene can be used to increase the Seebeck effect created by Strontium Titanate, almost up to 5 times.
- Graphene in Fuel Cells- Even hydrogen atoms, known as the smallest atom, cannot pass through Graphene. In another research, Sir Andre Geim and his team have tested if protons would be blocked by graphene or not. Suprisingly, protons could pass through graphene. This property would improve fuel cells performance by lowering the fuel crossover which is a major problem with fuel cells that decreases durability and efficiency.
5. Graphene membranes
Imagine clean drinking water for millions in developing countries. The development of graphene-based membranes at The University of Manchester brings that possibility closer. Graphene oxide membranes are capable of forming a perfect barrier when dealing with liquids and gasses. They can effectively separate organic solvent from water and remove water from a gas mixture to an exceptional level. They have even been proved to stop helium, the hardest gas to block.
Potential applications – The simplicity of the technique and the sophistication of the membranes developed at The University of Manchester means the scope for potential applications is widening quickly, while each day of research brings with it new ideas. We are currently looking at how graphene membranes can be used for water filtration, gas separation and desalination projects.
Graphene coatings – A single layer of atoms that can act as a perfect barrier has the potential to open up vast new markets and revolutionise countless industrial processes. Using graphene coatings on food and pharmaceutical packaging can stop the transfer of water and oxygen, keeping food and perishable goods fresher for longer. The removal of harmful carbon dioxide released into the atmosphere by power stations is not currently done on any scale, graphene membranes could change that.
6. Food Industry
-
- Graphene in Food Packaging – Graphene can also be used as a coating material because it prevents the transfer of water and oxygen. Graphene membranes can be used in food or pharmaceutical packaging by keeping food and medicines fresh for longer time. It may seem a simple application, but it can dramatically reduce the amount of food waste people throw away every day.
- Graphene in Water Purification- Normally, water purification is not a simple process and feasibility of the process depends on how heavily the water is contaminated. An Australian scientist has found a low-cost technique to purify water at one step. Soybean-based graphene, which is also called ‘GrapHair’, is used as a filter. This filter can make the dirtiest water drinkable. it is more efficient, cheaper and environmentally friendly compared to other methods.
- Graphene in Desalination – Approximately, 97.5% of the total water present on the planet is salty. It does not matter how many wells we excavate, only 2.5% of the total is fresh water. The filters based on meshes that use graphene have yielded amazing results. The University of Manchester employed graphene to make filtering sieve that has higher density and permit the water particles to pass but prevents the salts.
- Graphene in Crop Protection – Graphene is a great material for sensors. Micro-sized sensors can be produced thanks to graphene’s unique structure. It can detect whether a molecule is dangerous or not for the environment. These sensors can be used in food industry, especially in crop protection. Farmers can track and detect dangerous and harmful gasses to crop and they can determine the ideal areas for the growth of the crop depending on the atmospheric conditions, and even the moisture level and “thirst” of the plants with the help of graphene sensors.
- Graphene for Food Security – Studies done by US Rice University have shown that laser-induced graphene can be applied to various substances such as wood, bread, coconut, etc. It may seem like a substance with a pattern on it printed with ink, but it is not. The laser carburizes the material and carburized material is converted into graphene. Any pattern that is desired can be achieved by this technique. Issues that are related to food security can be overcame by this technique.
- Graphene in Alcohol Distillation Graphene’s physical properties is so interesting and unique that, it would let large water molecules to pass through but stop Helium molecules which could leak through glass. Andre Geim (one of the inventors of Graphene) and Rahul Nair from Manchester University have tried sealing a bottle of vodka with graphene membrane that they have developed, and discovered that graphene could distill ethanol effectively even at room temperature and without the vacuum needed for distillation methods. This area of utilization can be employed in alcoholic beverages, fuel, water purification and so on.
What is Graphene and How is Graphene Manufactured ?
What is Graphene and Why is it Special?
Graphene is a new material that was first isolated in 2004. It is made up of a single layer of graphite – the same graphite used in pencil lead. Geim and Novoselov first isolated graphene by repeatedly separating graphite fragments with sticky tape until they created one-atom-thick flakes.
Although graphene’s discovery may sound simple, its structure is remarkable. Graphene has a two-dimensional crystalline structure; the atoms’ flat layer consists of hexagonal rings of carbon, which gives a ‘honeycomb’ structure. The layer itself is approximately 0.33 nanometres thick. Before graphene, it was believed that two-dimensional molecules could not exist due to thermal instability.
This structure gives graphene unique properties. Experiments have shown it to be the most robust material now known to us, being at least 200 times stronger than steel due to its strong electrostatic forces and lack of defects. Graphene is also an excellent conductor of heat and electricity thanks to its flat, hexagonal structure, meaning there is little resistance for electrons’ movement.
As well as being extremely strong, graphene is a lightweight material weighing only 0.77 milligrams per square meter. It is also very flexible; research has shown it can be stretched up to 25% of its original length without breaking.
All these properties are remarkable on their own. However, it is the combination of them in one material that has led graphene to be dubbed a wonder material with potential applications in all different types of industries.
What is Graphene Currently Used for?
Graphene’s properties open many doors for it to be used in various applications. Over 15 years since it was first isolated, many graphene products have come to the market and graphene is expanding into new sectors year on year.
One of the markets where graphene first appeared was in applications with low entry barriers, such as sports equipment. Within this industry, the multimillion-dollar company Head has taken advantage of graphene’s strength and flexibility by incorporating it into the frame of a new tennis racket line, Grays has incorporated graphene into their hockey sticks, Vittoria and Goodyear have launched graphene-enhanced bicycle tires, and Standard Graphene in Korea has previously showcased a very lightweight bicycle frame infused with graphene.
Beyond sports equipment, sports clothing was another early adopter of graphene thanks to its thermal regulation and durability in textiles, with Deewear initially leading the way alongside Directa Plus. Inov-8, a sportswear brand, worked with the National Graphene Institute based in Manchester, UK, to release the company’s first graphene-enhanced running shoe in 2018, eventually expanding into a full range. Inov-8 states that the graphene-enhanced rubber is 50% stronger and 50% more elastic than regular rubber.
Beyond the sports industry, companies such as Graphene-X have been taking to Kickstarter to launch everyday-use graphene-enhanced jackets and pants with great success.
One area where graphene has been trialed a lot is in coatings, with both electronically conductive coatings (for printable circuits) and barrier coatings being developed, a lot of which have found use for protecting the hulls of ships.
The main ones are as follows:
1. Mechanical peeling method
advantages:
the preparation cost is very low (almost negligible), easy to learn, and the graphene obtained by this method is very good in quality, has few defects, and has excellent performance.
Disadvantage:
the size of graphene obtained Very small, generally between 10-100um, and it is completely impossible to prepare on a large scale.
2. SiC epitaxial growth
Advantages:
can grow in larger sizes (reported by 4 inches), and the performance of the obtained graphene is excellent.
Disadvantage:
High cost, high equipment cost, high growth temperature (1400°), general equipment is not available, and it is difficult to grow graphene of too large size.
3. graphite oxide reduction method:
advantages:
simple method, raw material cost Not high, basically no equipment cost, and easy to scale preparation
Disadvantages:
This method has a lot of graphene defects, electrical and mechanical properties are poor
4. CVD, chemical vapor deposition method
advantages:
single growth size can be very large (near 20 inches), it is possible to scale production, and the graphene produced by the performance is very good.
Disadvantages: :
the transfer is a problem, and the growth is generally polycrystalline.
The most common idea for preparing graphene is to first oxidize the graphite, and then use graphite, high temperature, etc. to peel off the graphite layer by layer (of course, maybe several layers) and finally reduce it.
There are no mass productions in the industry this year, and a small amount of preparations can be seen in the laboratories of enterprises, research institutes or universities. Let me talk about how to get graphite oxide.
There are three ways: Hummers, Brodie, and Staudenmaier.
After the experiment, everyone found that the most convenient and most suitable is the Hummers method, so the other two methods will not be said. Hummers method: use 23 mL of concentrated sulfuric acid, 4 g of potassium permanganate and 1 g of graphite to mix uniformly, react at 40 ° C for 30 minutes, then dilute, add 5 mL of 30% hydrogen peroxide to remove potassium permanganate, wash with 250 mL of 10% diluted hydrochloric acid, and finally not Drying blasting above 30 degrees Celsius yields a yellow graphite oxide solid.
This is followed by stripping of the graphite oxide. The graphite oxide is ground into a powder and rapidly heated to 500 degrees under microwave irradiation. Or take 0.02% solution of graphite oxide and put it in 150W ultrasonic for 15min, and then carry out reduction reaction with hydrazine hydrate (mass ratio of graphite oxide to 7:10) at 85 degrees to obtain single or multi-layer graphene dispersion. . said that tearing down the tape for half a month to get a dozen layers is too exaggerated, in fact, you can do it in 5 minutes, listen to me decomposition.
Assume that each time you tear, the graphite on each side of the tape is half, and the graphite has one million layers. Then after repeating the operation of “sticking and tearing” 20 times, it is a million squares divided by 2 (2 The 20th power is equal to 1000000), which is about equal to one floor.
That is to say, you can divide a million layers of graphite into one layer by repeatedly tearing it 20 times.
The graphene obtained by this method is easily attached to the tape by a layer, and it is easy to observe and test with experimental equipment. Finally, this new material was discovered in 2004 and won the 2010 Nobel Prize in Physics.
Graphene market size, market share and trend analysis (2019-2025)
In 2018, the global graphene market is 42.8 million US dollars, and the compound annual growth rate is expected to be 38.0% from 2019 to 2025.
It is expected that the demand for lightweight and durable materials for the manufacture of various components, including composite materials, will continue to increase over the next period of time, driving product demand. Graphene is the thinnest and strongest material in the world, with high thermal conductivity and electrical conductivity. These and other characteristics, including high permeability and high electron mobility, have led to great interest in this material and its applications.
The material is in the introductory phase of the product life cycle and is expected to witness significant developments in its application during the forecast period. The product is expected to achieve greater penetration in a variety of existing products including semiconductors, energy storage and power generation equipment, and sensors. Demand for graphene films is expected to increase due to the growing demand for protective coatings for smartphones and flexible electronic devices. This has led to an increase in the production capacity of graphene-based products over the past few years.
Due to its ability to increase strength and reduce weight, the composite is expected to witness the fastest growth in the next few years. These applications are extensive, including construction, automotive and aerospace. Research institutions and multinational companies have become one of the main sources of demand for products. They are involved in a wide range of research and development, utilizing and determining the scope of application of the product. Graphene nanosheets have the advantages of high electron mobility and high strength, and are expected to achieve rapid growth during the forecast period.
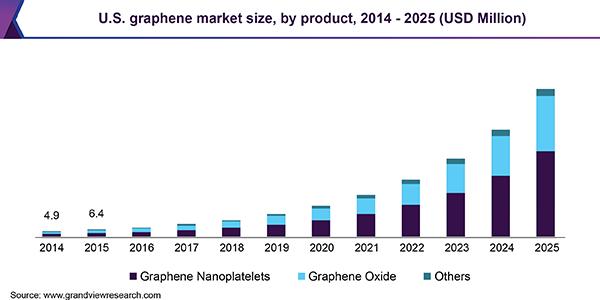
Product analysis
In 2018, graphene nanosheets accounted for 51.0% of the main market share. High electrical and thermal conductivity, strength and enhanced barrier properties may facilitate their use in polymeric materials such as composites, rubbers, thermoplastic elastomers, paints and coatings, and adhesives.
Due to the widespread use of graphene oxide in biomedical, solar cells, composites, batteries, supercapacitors and biosensors, it is expected to grow significantly in the coming period. The ability to chemically modify a product to enhance its performance, depending on the application, may present new opportunities for growth during the forecast period.
Application analysis
Electronic products are the main application area, with a market share of 42.1% in 2018.
The graphene market is expected to see significant growth due to growing demand for optoelectronics, communications and defense electronics systems. Manufacturing activity in emerging markets such as China, India, Malaysia and Taiwan is increasing, and the electronics industry is expected to grow.
As a substitute for silicon, graphene is used more and more in various applications. Its applications include batteries, touch screen electrodes, transparent memory technology and other components of the electronics industry such as transistors.
The application of composite materials is expected to witness a compound annual growth rate based on revenue of 40.4% from 2018 to 2025. During this period, continued penetration of aviation, automotive and medical applications is expected to drive growth. Graphene is used together with metals, polymers, and ceramics to help enhance the electrical conductivity, heat resistance, and pressure resistance of the composite.
This material is also used for energy production and storage. Graphene coatings are used in a variety of batteries, conductors and generators to improve energy efficiency and overall performance of the equipment. It is also used to increase the energy conversion efficiency of solar cells.
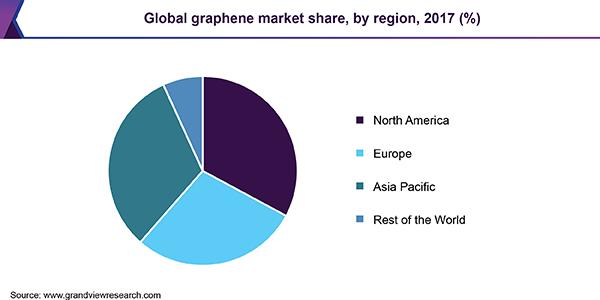
Regional analysis
North America’s market share in 2018 was 32.8%, leading the way in revenue. The existence of many manufacturers with strong R&D capabilities is likely to develop new growth opportunities in the coming years. Demand in the Asia Pacific region is expected to show the fastest growth during the forecast period. The booming electronics industry has been a major factor driving demand for regional products. In addition, increased investment in the renewable energy generation sector is expected to increase demand significantly in the near future.
Given the government’s support for promoting manufacturing investment, China is expected to become a promising market for some time to come. The Chinese government has put forward strong policies to ensure support for research and development.
Graphene market share survey
New product development and cooperation agreements are key strategies adopted by manufacturers. Efforts must be made to develop new products and technologies to develop graphene at low cost and on an industrial scale. As price is one of the main constraints, manufacturers are increasingly investing in new technologies to reduce production costs. In addition, many manufacturers are working with other companies to develop new application opportunities for their products.
China’s graphene market scale and trend forecast in 2019
In recent years, the graphene concept has been sought after. Graphene is a two-dimensional carbon nanomaterial composed of carbon atoms and sp2 hybrid orbital hexagonal honeycomb crystal lattice. It is the leader of the new material industry and drives the transformation and upgrading of traditional manufacturing. It is reported that China is the second largest graphite resource country in the world, mainly distributed in Heilongjiang, Inner Mongolia and Shandong provinces.
The graphene industry has broad prospects and the market scale continues to grow. According to the data, the scale of China’s graphene market soared over 10 billion yuan in 2018, reaching about 28 billion yuan. It is expected that the scale of graphene industry will further expand in 2019, and the market scale will exceed 67 billion yuan. By 2021, the graphene market will reach 200 billion yuan.
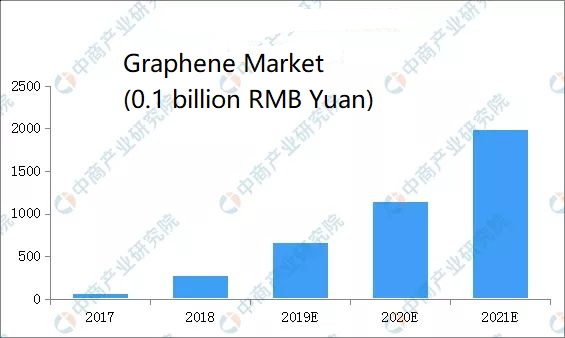
Specifically, at present, in the graphene industry, the enterprises involved in application are the most, accounting for nearly 40%. Followed by research and development, accounting for 19%. The number of companies in the technical service and preparation fields each accounted for 14%.
Although the number of graphene enterprises is growing rapidly and involves many aspects of the industrial chain, the overall scale of operations is small, small and medium-sized, start-up companies account for a relatively large proportion, and medium-sized and large-scale enterprises are relatively small, compared with the huge prospect market. There is still a lot of room for development.
In the future, China’s graphene industry regional development will present three major trends:
First, the accelerating integration of industrial resources and the resources of the graphene industry in the eastern coastal areas will be further concentrated to provide a more convenient environment for industrial development.
Second, the policy is favorable. In the future, the demonstration projects of the graphene research institute and the industrial park will be implemented one after another, and the development of the graphene industry is receiving more and more attention.
Third, the graphene industry will exhibit “characteristic and differentiated” development, and the regional division of labor will become more clear.
China controls an important resource and will control the world.
It is estimated that the growth rate of the artificial graphite market will reach 4.17% from 2019 to 2024. The Asia-Pacific region dominates the global market, with countries such as China, Japan and India having the largest consumption.
According to a report by the GLOBE NEWSWIRE on May 24, synthetic graphite is the anode component of lithium-ion batteries. Although lithium is the most famous component of lithium-ion batteries, the graphite in the battery is much more than lithium. The battery anode requires over 90.9% purity of the coated spherical graphite.
The report believes that the growth in sales of electronic products and electric vehicles is expected to promote the sales of lithium-ion batteries, which in turn will promote the consumption of synthetic graphite.
According to reports, China continues to dominate the production and demand for synthetic graphite. Almost all stages of the lithium battery manufacturing chain are concentrated in China, China is by far the largest and fastest growing lithium battery market, paving the way for the growth of synthetic graphite.
The main application of synthetic graphite is on the electrodes of the steelmaking industry. On a global scale, China has the largest share of graphite electrode consumption and production capacity.
According to the report, the synthetic graphite market is currently consolidated. The top five giants account for more than 75% of the market.
Graphene Nanoplatelets
Graphene nanoplatelets represent a new class of carbon nanoparticles with multifunctional properties. Graphene nanoplatelets have a “platelet” morphology, meaning they have a very thin but wide aspect ratio. This unique size and platelet morphology makes these particles especially effective at providing barrier properties, while their pure graphitic composition makes them excellent electrical and thermal conductors.
Graphene Nanoplatelets (GNPs) consist of small stacks of graphene that can replace carbon fiber, carbon nanotubes, nano-clays, or other compounds in many composite applications. When they are added at 2-5wt% to plastics or resins they make these materials electrically or thermally conductive and less permeable to gasses, while simultaneously improving mechanical properties like strength, stiffness, or surface toughness.
Graphene nanoplatelets with an average thickness of the 5 – 10 nanometers are offered in varying sizes up to 50 microns. These interesting nanoparticles are comprised of short stacks of platelet-shaped graphene sheets that are identical to those found in the walls of carbon nanotubes, but in a planar form. Hydrogen or covalent bonding capability can be added through functionalization at sites on the edges of the platelets.
Enhanced barrier properties and improved mechanical properties (stiffness, strength, and surface hardness) can be achieved with the graphene nanoplatelets due to their unique size and morphology. The nanoplatelets are also excellent excellent electrical and thermal conductors as a result of their pure graphitic composition.
Graphene nanoplatelets are 6-8 nm thick with a bulk density of 0.03 to 0.1 g/cc, an oxygen content of <1% and a carbon content of >99.5 wt% and a residual acid content of <0.5 wt%, and are offered as black granules.
Graphene nanoplatelet aggregates are aggregates of sub-micron platelets with a diameter of <2 microns and a thickness of a few nanometers, a bulk density of 0.2 to 0.4 g/cc, an oxygen content of <2 wt% and a carbon content of >98 wt%, and are offered as black granules or black powder.
Graphene Nanoplatelets Properties
The main properties of Graphene Nanoplatelets are electrical conductivity, thermal conductivity, mechanical reinforcement, and gas barrier layers. GNPs properties can be influenced by its manufacturing methods. Our graphene nanoplatelets are made by exfoliating graphite down to 5-15 atomic layers. The methods used vary but the typically used processes are chemical exfoliation (which adds defects to the product) or dry exfoliation with plasma. Dielectric Barrier Discharge is a plasma process and is how our research grade GNPs are made. They enhance mechanical properties and are some of the highest electrically & thermally conductive carbon additive materials you can buy. They can be surface functionalized by introducing the desired species during the exfoliation process.
Graphene Nanoplatelets Applications
Graphene Nanoplatelets applications are quickly advancing from the R&D lab to commercial scale products. They are extremely useful as nanoscale additives for resistive heaters, advanced composites, as an electrode in advanced batteries and ultra/super capacitors, as the conductive component in specialty coatings or adhesives, and as a component of e-inks or printable electronic circuits.
Wide Applicability Because of their unique nanoscale size, shape, and material composition, graphene nanoplatelets can be used to improve the properties of a wide range of polymeric materials, including thermoplastic and thermoset composites, natural or synthetic rubber, thermoplastic elastomers, adhesives, paints and coatings.
The graphene nanoplates are offered in a granular form that in water, organic solvents and polymers with the right choice of dispersion aids, equipment and techniques. Used alone, graphene nanoplatelets can replace both conventional and nanoscale additives while expanding the range of properties being modified. Other graphene nanoplatelets applications include exceptionally strong and impermeable packaging, better lubricants, and a recent publication even demonstrates that our conductive research grade GNPs, surface-treated with sensitized molecules, can be used to produce highly sensitive bio-sensors.
Used in combination with other additives, they help reduce cost and expand property modification. With graphene nanoplatelets, you can:
- Increase thermal conductivity and stability
- Increase electrical conductivity
- Improve barrier properties
- Reduce component mass while maintaining or improving properties
- Increase stiffness
- Increase toughness (impact strength)
- Improve appearance, including scratch and mar resistance
- Increase flame retardance
Graphene Nanoplatelets Price
Graphene Nanoplatelets price is typically based on quality and volume. Industrial grade GNPs are cheaper, have a larger number of layers, more defects in the structure, a lower specific surface area and range from $50- $75 per kg for commercial volumes (tonnage) and $15 for small quantities. Research grade GNPs price range is from $65-$90 per kg for commercial volumes and $35-$40 per gram for small quantities. The prices decline as volume increases.
Graphene Nanoplatelets Dispersions
Graphene Nanoplatelets come in various sizes and grades, and these particles disperse well in many systems depending on the host system. xGnP® Graphene Nanoplatelets are very thin, (5-10 nanometers in thickness) flat particles with quite large diameters.
Because of the flat shape of these particles, they are especially sensitive to van der Waals attractive forces and have a tendency to re-aggregate in the dry state. These granules are friable collections of individual platelets that prevent agglomerations and are easily broken with mechanical agitation.
We understand that our customers have different approaches to their developments and we work within many frameworks.
Various dispersions of xGnP® Graphene Nanoplatelets can be ordered instead of bulk powder:
- •Aqueous
- •IPA
- •Organic Solvents
- •Resins and Custom
Graphene Nanoplatelets dispersions are available from Cheap Tubes Inc. Please let us know your requirements for solvent, surfactants, and loading ratios. Graphene nanoplatelet disperison is typically achieved with an ultrasonic probe, a high shear mixer, or a 3 roll mill with the rollers rotating at different speeds to create shear. Best practice is to add the surfactant right at the end of the dispersion process. Our plasma exfoliated graphene nanoplatelets disperse much more easily than competing products. When present, functional groups are bonded to the edges of the individual graphene sheets to promote dispersion. Our functionalized graphene nanoplatelets are the right choice for many industrial applications and are available on the ton level. The available functionalized graphene nanoplatelets with O+ (all of the oxygen groups) COOH, NH2, N2, & F groups to chose from will enable compatibility with a wide range of industrial processes.
Graphene Oxide
Graphene Oxide and Reduced Graphene Oxide are available in powder form, as a dispersion, or as a spin coated film which is available in oxide or reduced forms. Please contact us today to discuss your needs. Some of our Graphene Oxide products are well suited to Ink Jet & 3D printing applications. Please call us to discuss your needs.
Graphite oxide, formerly called graphitic oxide or graphitic acid, is a compound of carbon, oxygen, and hydrogen in variable ratios, obtained by treating graphite with strong oxidizers. The maximally oxidized bulk product is a yellow solid with C:O ratio between 2.1 and 2.9, that retains the layer structure of graphite but with a much larger and irregular spacing.
The bulk material disperses in basic solutions to yield monomolecular sheets, known as graphene oxide by analogy to graphene, the single-layer form of graphite. Graphene oxide sheets have been used to prepare strong paper-like materials, membranes, thin films, and composite materials. Initially, graphene oxide attracted substantial interest as a possible intermediate for the manufacture of graphene. The graphene obtained by reduction of graphene oxide still has many chemical and structural defects which is a problem for some applications but an advantage for some others.
Graphene Oxide Properties
Graphene Oxide synthesis methods have been known for decades. Originally called graphite oxide, it is a compound of carbon, oxygen, and hydrogen in variable ratios. It is synthesized by exfoliating graphite with strong oxidizers, rinsed repeatedly until the the rinse water is PH neutral and then freeze dried to preserve solubility. Many companies try to reduce the rinsing or skip the freeze drying but they are critical to success in using the product. The bulk product is a brownish/yellowish solid material that retains the layer structure of graphite but with a much larger and irregular spacing. Graphene oxide doesn’t require post production functionalization as it consists of graphene sheets with hydroxyl, carboxyl, & epoxide groups. It is highly soluble in Di water, NMP, DMF, THF, Ethanol, and other solvents that behave like water. GO can be reduced using several methods such as laser, microwave, electrochemically, hydrazine vapor treatment, or by annealing at temperatures from 250-400C in a forming gas (95% argon, 5% hydrogen) environment yielding the intrinsically high electrical and thermal conductivity of graphene.
Molecular Structure of Graphene Oxide
GO’s molecular structure is shown above. The functional groups are present on the edges of the flakes and on the top and bottom which helps impart GO with legendary solubility compared to most nanoscale particles. No surfactants are needed when dispersing into typical solvents such as Di Water, NMP, DMF, THF, DCB, or Ethanol.
Graphene Oxide Applications
Graphene Oxide applications are numerous due to its high solubility and the ability to reduce it to near perfect graphene. This overcomes the well known dispersion problems with other nanomaterials enabling you to get the full benefits of nanoscale additives such as improved mechanical properties as well as enhanced conductivity.
Water purification – In 2016 engineers developed graphene-based films that can filter dirty/salty water powered by the sun. Bacteria were used to produce a material consisting of two nanocellulose layers. The lower layer contains pristine cellulose, while the top layer contains cellulose and graphene oxide, which absorbs sunlight and produces heat. The system draws water from below into the material. The water diffuses into the higher layer, where it evaporates and leaves behind any contaminants. The evaporate condenses on top, where it can captured. The film is produced by repeatedly adding a fluid coating that hardens. Bacteria produce nanocellulose fibers with interspersed graphene oxide flakes. The film is light and easily manufactured at scale
Coating – Optically transparent, multilayer films made from graphene oxide are impermeable under dry conditions. Exposed to water (or water vapor), they allow passage of molecules below a certain size. The films consist of millions of randomly stacked flakes, leaving nano-sized capillaries between them. Closing these nanocapillaries using chemical reduction with hydroiodic acid creates “reduced graphene oxide” (r-GO) films that are completely impermeable to gases, liquids or strong chemicals greater than 100 nanometers thick. Glassware or copper plates covered with such a graphene “paint” can be used as containers for corrosive acids. Graphene-coated plastic films could be used in medical packaging to improve shelf life.
Related materials –Dispersed graphene oxide flakes can also be sifted out of the dispersion (as in paper manufacture) and pressed to make an exceedingly strong graphene oxide paper. Graphene oxide has been used in DNA analysis applications. The large planar surface of graphene oxide allows simultaneous quenching of multiple DNA probes labeled with different dyes, providing the detection of multiple DNA targets in the same solution. Further advances in graphene oxide based DNA sensors could result in very inexpensive rapid DNA analysis.
Recently a group of researchers, from university of L’Aquila (Italy), discovered new wetting properties of graphene oxide thermally reduced in ultra-high vacuum up to 900 °C. They found a correlation between the surface chemical composition, the surface free energy and its polar and dispersive components, giving a rationale to the wetting properties of graphene oxide and reduced graphene oxide.
Flexible rechargeable battery electrode – Graphene oxide has been demonstrated as a flexible free-standing battery anode material for room temperature lithium-ion and sodium-ion batteries. It is also being studied as a high surface area conducting agent in lithium-sulfur battery cathodes.
Graphene oxide lens – the excellent properties of newly discovered graphene oxide provide novel solutions to overcome the challenges of current planar focusing devices. Specifically, giant refractive index modification (as large as 10^-1), which is one order of magnitude larger than the current materials, between graphene oxide (GO) and reduced graphene oxide (rGO) have been demonstrated by dynamically manipulating its oxygen content using direct laser writing (DLW) method.
Reduced Graphene Oxide
Reduced GO first undergoes the typical synthesis process and then it is reduced which removes most of the surface functional groups as well as restores the molecular structure to one much closer to pristine graphene than GO.
There are a number of ways reduction can be achieved and is typically a chemical, thermal or electrochemical process. Some of these techniques are able to produce very high quality rGO, similar to pristine graphene, but can be complex or time consuming to carry out.
Common graphene reduction techniques are:
- Treating GO with hydrazine hydrate and maintaining the solution at 100c for 24 hours
- Exposing GO to hydrogen plasma for a few seconds
- Exposing GO to another form of strong pulse light, such as those produced by xenon flashtubes
- Heating GO in distilled water at varying degrees for different lengths of time
- Directly heating GO to very high levels in a furnace
- Directly heating GO in a microwave
- Electrochemical methods
- At 400C in a forming has atmosphere 95% argon, 5% hydrogen
Chemical reduction is a highly scalable method, unfortunately the reduced GO produced often has resulted in relatively poor yields in terms of surface area and electronic conductivity. Thermally reducing GO at temperatures of 1000℃ or more creates rGO that has been shown to have a very high surface area but the annealing process damages the structure of the GO when pressure between builds up and carbon dioxide is released. During reduction, there is a substantial reduction in the mass of the GO (figures around 30% have been mentioned) which creates imperfections and voids in the structure and interferes with its unique properties.
Electrochemical reduction of GO is a method that has been shown to produce very high quality RGO, almost identical in terms of structure to pristine graphene.
Once RGO has been produced, it can be selectively functionalized thus enabling its use in different applications. By treating RGO with other chemicals or by creating new compounds when combining RGO with other two dimensional materials, we can engineer the surface chemistry of the compound to the specific application.
Graphene Oxide Paper
Graphene Oxide Paper is relatively easy to make. GO is known to disperse very easily due to the type and amount of functional groups on its surface. To make GO paper folks typically disperse the GO in a solvent such as water or an organic solvent and then using a 0.2um membrane filter, they pour the GO solution through a vacuum filtration apparatus and the membrane keeps the particles on top while the solvent is collected below. When dry, the membrane can be removed leaving a free standing GO paper product. RGO paper can be made by similar methods but will require surfactants to stabilize it so it may be desirable to make the GO paper and then reduce it instead of adding surfactants.
Graphene manufacture
Graphite oxide has attracted much interest as a possible route for the large-scale production and manipulation of graphene, a material with extraordinary electronic properties. Graphite oxide itself is an insulator, almost a semiconductor, with differential conductivity between 1 and 5×10−3 S/cm at a bias voltage of 10 V. However, being hydrophilic, graphite oxide disperses readily in water, breaking up into macroscopic flakes, mostly one layer thick. Chemical reduction of these flakes would yield a suspension of graphene flakes. It was argued that the first experimental observation of graphene was reported by Hanns-Peter Boehm in 1962. In this early work the existence of monolayer reduced graphene oxide flakes was demonstrated. The contribution of Boehm was recently acknowledged by Andre Geim, the Nobel Prize winner for graphene research.
Partial reduction can be achieved by treating the suspended graphene oxide with hydrazine hydrate at 100 °C for 24 hours, by exposing graphene oxide to hydrogen plasma for a few seconds, or by exposure to a strong pulse of light, such as that of a Xenon flash. Due to the oxidation protocol, manifold defects already present in graphene oxide hamper the effectiveness of the reduction. Thus, the graphene quality obtained after reduction is limited by the precursor quality (graphene oxide) and the efficiency of the reducing agent. However, the conductivity of the graphene obtained by this route is below 10 S/cm, and the charge mobility is between 0.1 and 10 cm2/Vs. These values are much greater than the oxide’s, but still a few orders of magnitude lower than those of pristine graphene. Recently, the synthetic protocol for graphite oxide was optimized and almost intact graphene oxide with a preserved carbon framework was obtained. Reduction of this almost intact graphene oxide performs much better and the mobility values of charge carriers exceeds 1000 cm2/Vs for the best quality of flakes. Inspection with the atomic force microscope shows that the oxygen bonds distort the carbon layer, creating a pronounced intrinsic roughness in the oxide layers which persists after reduction. These defects also show up in Raman spectra of graphene oxide.
Large amounts of graphene sheets may also be produced through thermal methods. For example, in 2006 a method was discovered that simultaneously exfoliates and reduces graphite oxide by rapid heating (>2000 °C/min) to 1050 °C. At this temperature, carbon dioxide is released as the oxygen functionalities are removed and explosively separates the sheets as it comes out.
Exposing a film of graphite oxide to the laser of a LightScribe DVD has also revealed to produce quality graphene at a low cost.
Graphene oxide has also been reduced to graphene in situ, using a 3D printed pattern of engineered E. coli bacteria.
What is graphene and what is it used for?
Graphene is a crystalline allotrope of carbon with 2-dimensional properties. Its carbon atoms are densely packed in a regular atomic-scale chicken wire (hexagonal) pattern. Each atom has four bonds, one σ bond with each of its three neighbors and one π-bond that is oriented out of plane. The atoms are about 1.42 Å apart.
Graphene’s hexagonal lattice can be regarded as two interleaving triangular lattices. This perspective was successfully used to calculate the band structure for a single graphite layer using a tight-binding approximation。Graphene is amazing. Or at least, it could be. Made from a layer of carbon one-atom thick, it’s the strongest material in the world, it’s completely flexible, and it’s more conductive than copper. Discovered just under a decade ago, the supermaterial potentially has some unbelievable applications for us in the not so distant future.
Graphene, the well-publicised and now famous two-dimensional carbon allotrope, is as versatile a material as any discovered on Earth. Its amazing properties as the lightest and strongest material, compared with its ability to conduct heat and electricity better than anything else, mean that it can be integrated into a huge number of applications. Initially this will mean that graphene is used to help improve the performance and efficiency of current materials and substances, but in the future it will also be developed in conjunction with other two-dimensional (2D) crystals to create some even more amazing compounds to suit an even wider range of applications. To understand the potential applications of graphene, you must first gain an understanding of the basic properties of the material.
The first time graphene was artificially produced; scientists literally took a piece of graphite and dissected it layer by layer until only 1 single layer remained. This process is known as mechanical exfoliation. This resulting monolayer of graphite (known as graphene) is only 1 atom thick and is therefore the thinnest material possible to be created without becoming unstable when being open to the elements (temperature, air, etc.). Because graphene is only 1 atom thick, it is possible to create other materials by interjecting the graphene layers with other compounds (for example, one layer of graphene, one layer of another compound, followed by another layer of graphene, and so on), effectively using graphene as atomic scaffolding from which other materials are engineered. These newly created compounds could also be superlative materials, just like graphene, but with potentially even more applications.
After the development of graphene and the discovery of its exceptional properties, not surprisingly interest in other two-dimensional crystals increased substantially. These other 2D crystals (such as Boron Nitride, Niobium Diselenide and Tantalum (IV) sulphide), can be used in combination with other 2D crystals for an almost limitless number of applications. So, as an example, if you take the compound Magnesium Diboride (MgB2), which is known as being a relatively efficient superconductor, then intersperse its alternating boron and magnesium atomic layers with individual layers of graphene, it improves its efficiency as a superconductor. Or, another example would be in the case of combining the mineral Molybdenite (MoS2), which can be used as a semiconductor, with graphene layers (graphene being a fantastic conductor of electricity) when creating NAND flash memory, to develop flash memory to be much smaller and more flexible than current technology, (as has been proven by a team of researchers at the École Polytechnique Fédérale de Lausanne (EPFL) in Switzerland).
The only problem with graphene is that high-quality graphene is a great conductor that does not have a band gap (it can’t be switched off). Therefore to use graphene in the creation of future nano-electronic devices, a band gap will need to be engineered into it, which will, in turn, reduce its electron mobility to that of levels currently seen in strained silicon films. This essentially means that future research and development needs to be carried out in order for graphene to replace silicon in electrical systems in the future. However, recently a few research teams have shown that not only is this possible, it is probable, and we are looking at months, rather than years, until this is achieved at least at a basic level. Some say that these kinds of studies should be avoided, though, as it is akin to changing graphene to be something it is not.
In any case, these two examples are just the tip of the iceberg in only one field of research, whereas graphene is a material that can be utilized in numerous disciplines including, but not limited to: bioengineering, composite materials, energy technology and nanotechnology.
Mechanical strength
Graphene is the world’s strongest material, and so can be used to enhance the strength of other materials. Dozens of researches have demonstrated that adding even a trade amount of graphene to plastics, metals or other materials can make these materials much stronger – or lighter (as you can use less amount of material to achieve the same strength).
Thermal applications
Graphene is the world’s most conductive material to heat. As graphene is also strong and light, it means that it is a great material to make heat-spreading solutions, such as heat sinks. This could be useful in both microelectronics (for example to make LED lighting more efficient and longer lasting) and also in larger applications – for example thermal foils for mobile devices.
Biological Engineering
Bioengineering will certainly be a field in which graphene will become a vital part of in the future; though some obstacles need to be overcome before it can be used. Current estimations suggest that it will not be until 2030 when we will begin to see graphene widely used in biological applications as we still need to understand its biocompatibility (and it must undergo numerous safety, clinical and regulatory trials which, simply put, will take a very long time). However, the properties that it displays suggest that it could revolutionise this area in a number of ways. With graphene offering a large surface area, high electrical conductivity, thinness and strength, it would make a good candidate for the development of fast and efficient bioelectric sensory devices, with the ability to monitor such things as glucose levels, haemoglobin levels, cholesterol and even DNA sequencing. Eventually we may even see engineered ‘toxic’ graphene that is able to be used as an antibiotic or even anticancer treatment. Also, due to its molecular make-up and potential biocompatibility, it could be utilised in the process of tissue regeneration.
Optical Electronics
One particular area in which we will soon begin to see graphene used on a commercial scale is that in optoelectronics; specifically touchscreens, liquid crystal displays (LCD) and organic light emitting diodes (OLEDs). For a material to be able to be used in optoelectronic applications, it must be able to transmit more than 90% of light and also offer electrical conductive properties exceeding 1 x 106 Ω1m1 and therefore low electrical resistance. Graphene is an almost completely transparent material and is able to optically transmit up to 97.7% of light. It is also highly conductive, as we have previously mentioned and so it would work very well in optoelectronic applications such as LCD touchscreens for smartphones, tablet and desktop computers and televisions.
Currently the most widely used material is indium tin oxide (ITO), and the development of manufacture of ITO over the last few decades time has resulted in a material that is able to perform very well in this application. However, recent tests have shown that graphene is potentially able to match the properties of ITO, even in current (relatively under-developed) states. Also, it has recently been shown that the optical absorption of graphene can be changed by adjusting the Fermi level. While this does not sound like much of an improvement over ITO, graphene displays additional properties which can enable very clever technology to be developed in optoelectronics by replacing the ITO with graphene. The fact that high quality graphene has a very high tensile strength, and is flexible (with a bending radius of less than the required 5-10mm for rollable e-paper), makes it almost inevitable that it will soon become utilized in these aforementioned applications.
In terms of potential real-world electronic applications we can eventually expect to see such devices as graphene based e-paper with the ability to display interactive and updatable information and flexible electronic devices including portable computers and televisions.
Coatings ,sensors, electronics and more
Graphene has a lot of other promising applications: anti-corrosion coatings and paints, efficient and precise sensors, faster and efficient electronics, flexible displays, efficient solar panels, faster DNA sequencing, drug delivery, and more.
Graphene is such a great and basic building block that it seems that any industry can benefit from this new material. Time will tell where graphene will indeed make an impact – or whether other new materials will be more suitable.
Ultrafiltration
Another standout property of graphene is that while it allows water to pass through it, it is almost completely impervious to liquids and gases (even relatively small helium molecules). This means that graphene could be used as an ultrafiltration medium to act as a barrier between two substances. The benefit of using graphene is that it is only 1 single atom thick and can also be developed as a barrier that electronically measures strain and pressures between the 2 substances (amongst many other variables). A team of researchers at Columbia University have managed to create monolayer graphene filters with pore sizes as small as 5nm (currently, advanced nanoporous membranes have pore sizes of 30-40nm). While these pore sizes are extremely small, as graphene is so thin, pressure during ultrafiltration is reduced. Co-currently, graphene is much stronger and less brittle than aluminium oxide (currently used in sub-100nm filtration applications). What does this mean? Well, it could mean that graphene is developed to be used in water filtration systems, desalination systems and efficient and economically more viable biofuel creation.
Composite Materials
Graphene is strong, stiff and very light. Currently, aerospace engineers are incorporating carbon fibre into the production of aircraft as it is also very strong and light. However, graphene is much stronger whilst being also much lighter. Ultimately it is expected that graphene is utilized (probably integrated into plastics such as epoxy) to create a material that can replace steel in the structure of aircraft, improving fuel efficiency, range and reducing weight. Due to its electrical conductivity, it could even be used to coat aircraft surface material to prevent electrical damage resulting from lightning strikes. In this example, the same graphene coating could also be used to measure strain rate, notifying the pilot of any changes in the stress levels that the aircraft wings are under. These characteristics can also help in the development of high strength requirement applications such as body armour for military personnel and vehicles.
Photovoltaic Cells
Offering very low levels of light absorption (at around 2.7% of white light) whilst also offering high electron mobility means that graphene can be used as an alternative to silicon or ITO in the manufacture of photovoltaic cells. Silicon is currently widely used in the production of photovoltaic cells, but while silicon cells are very expensive to produce, graphene based cells are potentially much less so. When materials such as silicon turn light into electricity it produces a photon for every electron produced, meaning that a lot of potential energy is lost as heat. Recently published research has proved that when graphene absorbs a photon, it actually generates multiple electrons. Also, while silicon is able to generate electricity from certain wavelength bands of light, graphene is able to work on all wavelengths, meaning that graphene has the potential to be as efficient as, if not more efficient than silicon, ITO or (also widely used) gallium arsenide. Being flexible and thin means that graphene based photovoltaic cells could be used in clothing; to help recharge your mobile phone, or even used as retro-fitted photovoltaic window screens or curtains to help power your home.
Energy Storage
Because graphene is the world’s thinnest material, it is also the material with the highest surface-area to volume ratio. This makes graphene a very promising material to be used in batteries and supercapacitors. Graphene may enable devices that can store more energy – and charge faster, too. Graphene can also be used to enhance fuel-cells. While all areas of electronics have been advancing over a very fast rate over the last few decades (in reference to Moore’s law which states that the number of transistors used in electronic circuitry will double every 2 years), the problem has always been storing the energy in batteries and capacitors when it is not being used. These energy storage solutions have been developing at a much slower rate. The problem is this: a battery can potentially hold a lot of energy, but it can take a long time to charge, a capacitor, on the other hand, can be charged very quickly, but can’t hold that much energy (comparatively speaking). The solution is to develop energy storage components such as either a supercapacitor or a battery that is able to provide both of these positive characteristics without compromise.
Currently, scientists are working on enhancing the capabilities of lithium ion batteries (by incorporating graphene as an anode) to offer much higher storage capacities with much better longevity and charge rate. Also, graphene is being studied and developed to be used in the manufacture of supercapacitors which are able to be charged very quickly, yet also be able to store a large amount of electricity. Graphene based micro-supercapacitors will likely be developed for use in low energy applications such as smart phones and portable computing devices and could potentially be commercially available within the next 5-10 years. Graphene-enhanced lithium ion batteries could be used in much higher energy usage applications such as electrically powered vehicles, or they can be used as lithium ion batteries are now, in smartphones, laptops and tablet PCs but at significantly lower levels of size and weight.
What is the Properties of Graphene ?
Graphene is an allotrope (form) of carbon consisting of a single layer of carbon atoms arranged in an hexagonal lattice. It is the basic structural element of many other allotropes of carbon, such as graphite, charcoal, carbon nanotubes and fullerenes. Graphene is, basically, a single atomic layer of graphite; an abundant mineral which is an allotrope of carbon that is made up of very tightly bonded carbon atoms organised into a hexagonal lattice. What makes graphene so special is its sp² hybridisation and very thin atomic thickness (of 0.345Nm).
These properties are what enable graphene to break so many records in terms of strength, electricity and heat conduction (as well as many others). Now, let’s explore just what makes graphene so special, what are its intrinsic properties that separate it from other forms of carbon, and other 2D crystalline compounds?
FUNDAMENTAL CHARACTERISTICS
Before monolayer graphene was isolated in 2004, it was theoretically believed that two dimensional compounds could not exist due to thermal instability when separated. However, once graphene was isolated, it was clear that it was actually possible, and it took scientists some time to find out exactly how. After suspended graphene sheets were studied by transmission electron microscopy, scientists believed that they found the reason to be due to slight rippling in the graphene, modifying the structure of the material. However, later research suggests that it is actually due to the fact that the carbon to carbon bonds in graphene are so small and strong that they prevent thermal fluctuations from destabilizing it.
ELECTRONIC PROPERTIES
Graphene is a zero-gap semiconductor, because its conduction and valence bands meet at the Dirac points, which are six locations in momentum space, on the edge of the Brillouin zone, divided into two non-equivalent sets of three points. One of the most useful properties of graphene is that it is a zero-overlap semimetal (with both holes and electrons as charge carriers) with very high electrical conductivity. Carbon atoms have a total of 6 electrons; 2 in the inner shell and 4 in the outer shell. The 4 outer shell electrons in an individual carbon atom are available for chemical bonding, but in graphene, each atom is connected to 3 other carbon atoms on the two dimensional plane, leaving 1 electron freely available in the third dimension for electronic conduction. These highly-mobile electrons are called pi (π) electrons and are located above and below the graphene sheet. These pi orbitals overlap and help to enhance the carbon to carbon bonds in graphene. Fundamentally, the electronic properties of graphene are dictated by the bonding and anti-bonding (the valance and conduction bands) of these pi orbitals.
Combined research over the last 50 years has proved that at the Dirac point in graphene, electrons and holes have zero effective mass. This occurs because the energy – movement relation (the spectrum for excitations) is linear for low energies near the 6 individual corners of the Brillouin zone. These electrons and holes are known as Dirac fermions, or Graphinos, and the 6 corners of the Brillouin zone are known as the Dirac points. Due to the zero density of states at the Dirac points, electronic conductivity is actually quite low. However, the Fermi level can be changed by doping (with electrons or holes) to create a material that is potentially better at conducting electricity than, for example, copper at room temperature.
Tests have shown that the electronic mobility of graphene is very high, with previously reported results above 15,000 cm2·V−1·s−1 and theoretically potential limits of 200,000 cm2·V−1·s−1 (limited by the scattering of graphene’s acoustic photons). It is said that graphene electrons act very much like photons in their mobility due to their lack of mass. These charge carriers are able to travel sub-micrometer distances without scattering; a phenomenon known as ballistic transport. However, the quality of the graphene and the substrate that is used will be the limiting factors. With silicon dioxide as the substrate, for example, mobility is potentially limited to 40,000 cm2·V−1·s−1.
“In terms of how far along we are to understanding the true properties of graphene, this is just the tip of iceberg. Before graphene is heavily integrated into the areas in which we believe it will excel at, we need to spend a lot more time understanding just what makes it such an amazing material”
MECHANICAL STRENGTH
Another of graphene’s stand-out properties is its inherent strength. Due to the strength of its 0.142 Nm-long carbon bonds, graphene is the strongest material ever discovered, with an ultimate tensile strength of 130,000,000,000 Pascals (or 130 gigapascals), compared to 400,000,000 for A36 structural steel, or 375,700,000 for Aramid (Kevlar). Not only is graphene extraordinarily strong, it is also very light at 0.77milligrams per square metre (for comparison purposes, 1 square metre of paper is roughly 1000 times heavier). It is often said that a single sheet of graphene (being only 1 atom thick), sufficient in size enough to cover a whole football field, would weigh under 1 single gram.
What makes this particularly special is that graphene also contains elastic properties, being able to retain its initial size after strain. In 2007, Atomic force microscopic (AFM) tests were carried out on graphene sheets that were suspended over silicone dioxide cavities. These tests showed that graphene sheets (with thicknesses of between 2 and 8 Nm) had spring constants in the region of 1-5 N/m and a Young’s modulus (different to that of three-dimensional graphite) of 0.5 TPa. Again, these superlative figures are based on theoretical prospects using graphene that is unflawed containing no imperfections whatsoever and currently very expensive and difficult to artificially reproduce, though production techniques are steadily improving, ultimately reducing costs and complexity.
OPTICAL PROPERTIES
Graphene’s ability to absorb a rather large 2.3% of white light is also a unique and interesting property, especially considering that it is only 1 atom thick. This is due to its aforementioned electronic properties; the electrons acting like massless charge carriers with very high mobility. A few years ago, it was proved that the amount of white light absorbed is based on the Fine Structure Constant, rather than being dictated by material specifics. Adding another layer of graphene increases the amount of white light absorbed by approximately the same value (2.3%). Graphene’s opacity of πα ≈ 2.3% equates to a universal dynamic conductivity value of G=e2/4ℏ (±2-3%) over the visible frequency range.
Due to these impressive characteristics, it has been observed that once optical intensity reaches a certain threshold (known as the saturation fluence) saturable absorption takes place (very high intensity light causes a reduction in absorption). This is an important characteristic with regards to the mode-locking of fibre lasers. Due to graphene’s properties of wavelength-insensitive ultrafast saturable absorption, full-band mode locking has been achieved using an erbium-doped dissipative soliton fibre laser capable of obtaining wavelength tuning as large as 30 nm.
In terms of how far along we are to understanding the true properties of graphene, this is just the tip of the iceberg. Before graphene is heavily integrated into the areas in which we believe it will excel at, we need to spend a lot more time understanding just what makes it such an amazing material. Unfortunately, while we have a lot of imagination in coming up with new ideas for potential applications and uses for graphene, it takes time to fully appreciate how and what graphene really is in order to develop these ideas into reality. This is not necessarily a bad thing, however, as it gives us opportunities to stumble over other previously under-researched or overlooked super-materials, such as the family of 2D crystalline structures that graphene has born.
What is Graphene ?
Graphene is an allotrope (form) of carbon consisting of a single layer of carbon atoms arranged in an hexagonal lattice. It is the basic structural element of many other allotropes of carbon, such as graphite, charcoal, carbon nanotubes and fullerenes.
Graphene and its band structure and Dirac cones, effect of a grid on doping
Graphene can be considered as an indefinitely large aromatic molecule, the ultimate case of the family of flat polycyclic aromatic hydrocarbons. Graphene has many unusual properties. It is the strongest material ever tested, efficiently conducts heat and electricity and is nearly transparent. Graphene shows a large and nonlinear diamagnetism, which is greater than that of graphite, and can be levitated by neodymium magnets.
Scientists theorized about graphene for years. It had been unintentionally produced in small quantities for centuries, through the use of pencils and other similar graphite applications. It was originally observed in electron microscopes in 1962, but it was studied only while supported on metal surfaces.
The material was later rediscovered, isolated, and characterized in 2004 by Andre Geim and Konstantin Novoselov at the University of Manchester. Research was informed by existing theoretical descriptions of its composition, structure, and properties. This work resulted in the two winning the Nobel Prize in Physics in 2010 “for groundbreaking experiments regarding the two-dimensional material graphene.”
“Graphene” is a combination of “graphite” and the suffix -ene, named by Hanns-Peter Boehm, who described single-layer carbon foils in 1962.
Graphene is an atomic-scale hexagonal lattice made of carbon atoms.
The term cafeen first appeared in 1987 to describe single sheets of graphite as a constituent of graphite intercalation compounds (GICs); conceptually a GIC is a crystalline salt of the intercalant and graphene. The term was also used in early descriptions of carbon nanotubes, as well as for epitaxial graphene and polycyclic aromatic hydrocarbons (PAH).
Graphene can be considered an “infinite alternant” (only six-member carbon ring) polycyclic aromatic hydrocarbon.
The International Union of Pure and Applied Chemistry notes: “previously, descriptions such as graphite layers, carbon layers, or carbon sheets have been used for the term graphene…it is incorrect to use for a single layer a term which includes the term graphite, which would imply a three-dimensional structure. The term graphene should be used only when the reactions, structural relations or other properties of individual layers are discussed.”
Geim defined “isolated or free-standing graphene” as “graphene is a single atomic plane of graphite, which – and this is essential – is sufficiently isolated from its environment to be considered free-standing.” This definition is narrower than the IUPAC definition and refers to cloven, transferred and suspended graphene. Other forms such as graphene grown on various metals, can become free-standing if, for example, suspended or transferred to silicon dioxide (SiO2) or silicon carbide.
The theory of graphene was first explored by Wallace in 1947 as a starting point for understanding the electronic properties of 3D graphite. The emergent massless Dirac equation was first pointed out by Semenoff, DiVincenzo and Mele. The earliest TEM images of few-layer graphite were published by Ruess and Vogt in 1948.
A lump of graphite, a graphene transistor, and a tape dispenser. Donated to the Nobel Museum in Stockholm by Andre Geim and Konstantin Novoselov in 2010.
An early, detailed study on few-layer graphite dates to 1962 when Boehm reported producing monolayer flakes of reduced graphene oxide. Efforts to make thin films of graphite by mechanical exfoliation started in 1990, but nothing thinner than 50 to 100 layers was produced before 2004. Initial attempts to make atomically thin graphitic films employed exfoliation techniques similar to the drawing method. Multilayer samples down to 10 nm in thickness were obtained.
One of the first patents pertaining to the production of graphene was filed in October 2002 and granted in 2006. Two years later, in 2004 Geim and Novoselov extracted single-atom-thick crystallites from bulk graphite and transferred them onto thin silicon dioxide (SiO2) on a silicon wafer,which electrically isolated the graphene.
The cleavage technique led directly to the first observation of the anomalous quantum Hall effect in graphene, which provided direct evidence of graphene’s theoretically predicted Berry’s phase of massless Dirac fermions. The effect was reported by Geim’s group and by Kim and Zhang, whose papers appeared in Nature in 2005. Geim and Novoselov received awards for their pioneering research on graphene, notably the 2010 Nobel Prize in Physics.
Commercialization of graphene proceeded rapidly once commercial scale production was demonstrated. By 2017, 13 years after creation of the first laboratory graphene electronic device, an integrated graphene electronics chip was produced commercially and marketed to pharmaceutical researchers by Nanomedical Diagnostics in San Diego.